Christoph Lohr: The water-energy nexus and water conservation
Misunderstanding Roy — Part 5

tothemoonphoto/iStock/Getty Images Plus via Getty Images.
Almost 80% of all water consumption comes from two groups: No. 1, thermoelectric power, and No. 2, irrigation (farming). Public supply, which includes industrial buildings, commercial buildings and residences, accounts for only 10%. When we have spoken about water conservation our conversation revolves around 10% of all usage. Therefore, as I argued in my last column, the answer to better water efficiency requires our society to address the water-energy nexus and smarter irrigation. To address the water-energy nexus, we are going to first address energy production, followed by evaluating the nexus itself. After addressing the water-energy nexus we will look at some of the agricultural uses of water. By reviewing our “Pareto Drivers” of water use, hopefully, we can gain a better context of how water conservation in the built environment impacts overall sustainability.
Energy production 101
The subject of energy production in the U.S. is one that has many voices. Many, if not most, of these voices are conflicting. To cover the entirety of all energy production would require an article the length of a book, so we will attempt to cover some of the basics for the purposes of this column.
According to the U.S. Energy Information Administration, a little less than 40% of our electricity comes from natural gas, while almost a quarter of electricity comes from coal. Both types of fuel not only release carbon (and other greenhouse gases) but also need a high amount of water in order to cool the steam that was used to power a turbine back into water.
All power plant design is more or less the same. A heat source (heat exchanger, coal furnace, etc.) heats water to the point that it becomes steam; the steam routes to a steam turbine, where the steam causes the turbine to spin very quickly. At the other end of the turbine (separated from the steam) are metallic bristles that brush against another metal side, which creates an electrical charge, and voila — you have electricity! Post-turbine, the steam (which has already partially condensed) goes to a condenser (another heat exchanger) which collects the steam and converts it back into water in order for it to be pumped back to the heat generator to repeat the cycle. (Natural gas power plants are a bit more efficient than coal power plants, as they utilize a gas turbine upstream of the heat exchanger to create additional electricity.) Cooling water is used to help cool the steam to pump it back to the steam generator. This condensing heat exchanger’s “condensing water” is taken to a cooling tower, which evaporates some of the water in the air to help lower the water temperature. This also means that water makeup is needed to replace the evaporated water. The heat source to create steam differs. The largest percentages of “heat source” historically have been coal and natural gas, but there is public pressure to reduce these fossil fuels and even eliminate them. While we will discuss this more later, one alternative heat source is nuclear power.
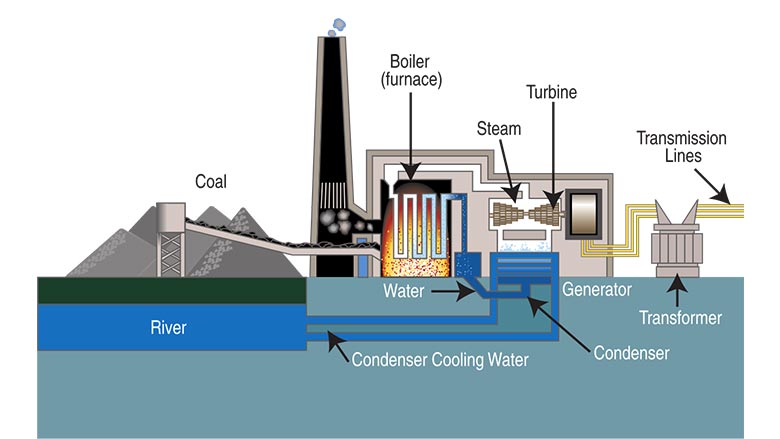
Nuclear power as a heat source for thermoelectric power generation has many advantages, starting with its fuel source: Uranium is one of the most energy-dense materials known to man. One pellet of uranium (which is as long as a quarter) is equivalent to 149 gallons of oil, 1 ton of coal or 17,000 cubic feet of natural gas. In the U.S., just under 20% of all electricity generation comes from 55 nuclear power plants. Additionally, the spent nuclear fuel can be recycled, using up even more energy. Unfortunately, concerns about nuclear waste and safety are rife with misinformation. When comparing various forms of energy, nuclear comes out as the safest form of energy time and time again. Finally, in terms of life cycle emissions, nuclear power has equal or less of a carbon footprint than many renewables.
The United Nations defines renewable energy as “energy that is derived from natural processes (e.g., sunlight and wind) that are replenished at a higher rate than they are consumed. Solar, wind, geothermal, hydro and biomass are common sources of renewable energy.” Renewable energy sources in many parts of the world make sense. I had the opportunity to visit Iceland years ago, which is powered 100% by geothermal heat. However, geothermal power is available in only a few geographic areas. Most frequently, renewable energy refers to wind and solar.
There are significant energy production limits for solar and wind. Both of these types of renewable energy sources are intermittent; that is, the wind dies down frequently and the sun does not shine at night. From a solar perspective, there are some parts of the U.S. that are abnormally sunny (like my home in Phoenix, Arizona) but other parts of the U.S. (like Portland, Oregon) are only sunny about a quarter of the time. Less sun equals less energy produced (and this occurs every night). For wind, actual productivity is typically much less than the rated capacity. This is an easy visualization: How many times have we driven by a wind farm and the blades are still? Wind turbine blades not turning equals no energy produced. No or less energy produced means more energy backup in the form of thermoelectric power or batteries. Additionally, from a water usage standpoint, solar panels (often but not always installed in deserts or other sunny places for the highest energy output) will lose capacity due to getting dirty, and therefore need washing from time to time to keep them operating at peak capacity. Hydropower is one of the oldest renewable energy sources. Capacity and reliability, however, of hydropower are becoming a concern. Take the Hoover Dam for example, where water scarcity on the Colorado River is becoming an energy issue as the dam requires at least 950 feet of water. The current water level on Lake Mead is only 1,040 feet. We could be looking at a situation where the Hoover Dam doesn’t produce power. In addition, as the water level lowers, the amount of power the dam produces will also decrease.
There is a belief that renewable energy resources such as solar, wind or hydro can replace traditional thermoelectric power sources. The problem is that renewable energy sources were never designed to be the majority of our power sources. At best, they can be a supplement energy source to more stable and powerful energy sources. To get the same amount of energy as a nuclear power plant, solar power requires 300 times more land than nuclear power. Wind would require almost 600 times more land than nuclear power. In order to decarbonize we need to turn to true engineering principles and away from political platitudes and a science-defying-faith that the laws of physics do not apply to energy production. From a scientific standpoint, if we want to reduce carbon emissions, the energy
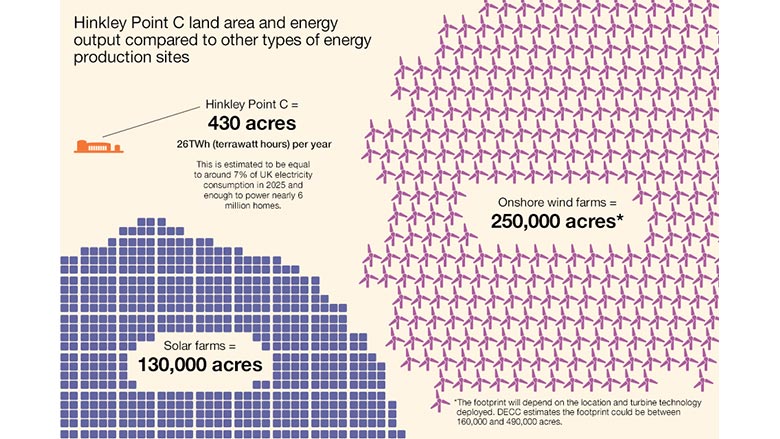
The unreliable nature of renewable energy is on full display in Germany. In 2019 I was shocked at the Frankfurt airport to see German magazine Der Spiegel with a cover article titled “Murks in Germany” — meaning “A Botched Job in Germany” in English. The cover showed broken wind turbines and electrical grid in front of a light-less Berlin. The cover was quite shocking because for most of the 2010s, German renewable energy was held up as the poster child of renewable energy success. In 2011, Germany decided to eliminate its last nuclear plants in favor of renewables in a knee-jerk reaction to the Fukushima Nuclear Power plant incident. Fast forward eight years and Germany’s decision to rely on renewable energy (titled Energiewende) was shown to be a failure. Even with a massive influx in solar panels and wind turbines, when the nuclear power plants were closed, coal power plants — not renewable energy sources — replaced them. In 2019, McKinsey forecasted that Energiewende also threatened Germany’s economy through decreasing the reliability of its energy supply. All of this occurred while Germans saw their electricity prices rise to triple that of nuclear-power-supplied-neighbor France. Fast forward to the present day, and Germany has to consider energy rationing or starting up more coal power plants due to its reliance on Russian natural gas-created electricity.
Batteries are commonly suggested as a way to help make unreliable renewables more reliable. The concern is doubling up of materials in order to make everything work. A more practical way may involve making something old new again: Hydrogen.
In September, I had the privilege of listening to a presentation at the IAPMO conference by SoCal Gas on hydrogen gas. The speaker asked an excellent question during his presentation: “Is it really sustainable to abandon in place the 100,000-plus miles of natural gas pipelines in California? Or is it better to find a way to repurpose them?” Of course repurposing these pipelines safely is the sustainable answer, and hydrogen gas created by renewables was the solution provided by SoCalGas; this makes sense. Another source of hydrogen gas could be nuclear power though — nuclear power typically maintains a consistent load and doesn’t ramp up/down very quickly. Using the “excess” nuclear energy to create hydrogen is a viable and resilient energy solution that, as my colleague John Mullen indicates, makes building electrification a possibility.
As plumbing engineers, we have to account for science and economics; in the realm of energy production and policy, it is the same. I have seen many claim that renewable energy costs are continually coming down; this is true. But there are also many who claim that renewables are less expensive than nuclear power; this is false. This falsehood of renewables being the least costly energy source becomes convoluted by incomplete at best (or misleading at worst) information.
The incomplete estimate of total energy cost is known as “Levelized Cost of Electricity” or LCOE. LCOE often gets used in the U.S. as a measure of energy efficiency. I’ve even seen some engineering firms that push for building electrification based on this faulty metric. LCOE includes cost of building, cost of fuel, cost of operation, and, sometimes, CO2 taxes. But even the U.S. Energy Information Administration notes the shortcomings of using LCOE. A better metric for estimating the total cost of energy is known as “Full Cost of Electricity” or FCOE, as documented in a peer-reviewed research paper titled “Full Cost of Electricity ‘FCOE’ and Energy Returns ‘eROI.’” In this paper, the “hidden” costs are revealed, which include cost of (electricity) transportation/balancing, cost of storage, cost of backup, cost of emissions, cost of recycling, room cost (sometimes called land footprint or energy sprawl) and other metrics (such as material input per unit of service, lifetime or energy return on investment). When these other types of metrics are utilized, it shows that, in terms of costs, the best energy return on investment is nuclear.
All of this lends itself to the case that renewable can’t power modern civilization. Some kind of reliable, energy-dense and cost-effective baseload is needed. Based on those criteria, there is one technology that fits the bill at this time: Nuclear energy. Not only would nuclear energy be able to provide the majority of the baseload, but in conjunction with renewables, they could create hydrogen gas, which could be used as a “battery” for peak demand.
In summary, there are three key engineering criteria when it comes to energy production: Energy density, reliability and cost. From the available options, the answer becomes pretty clear — in general, our transition as a nation should be towards utilizing nuclear power first and foremost, followed by some renewables with some kind of small “battery” backup. I would propose a breakdown of the following energy sources:
Water-energy nexus
Water and energy are intertwined. Civilizations typically need two foundational things to thrive: Water/sanitation and cheap and reliable energy delivery. Water plays an integral role in the development of energy, but water use is also a large consumer of energy. This is a bit of a “chicken-and-egg” situation. As mentioned in my last article, thermoelectric power generation utilizes a lot of water — about 40% of all freshwater use in the U.S. is used to create electricity. This primarily takes the form of steam coming off cooling towers. Water is likely used for manufacturing energy-thin renewable energy — (think of the FCOE material chart above), but water is also needed (albeit in smaller quantities) to keep renewables working — you need to wash solar panels or the dust reduces their energy output. With the sunniest places in the U.S. (like Arizona) also having high amounts of dust, this needs to be considered.
In order to minimize water usage for energy creation, wastewater reuse may be the solution. A great example is actually in my backyard of Phoenix, Arizona, where we have the Palo Verde Generating Station. This nuclear power plant produces a huge, huge number of megawatts of power every year with much of the power going to the city of Phoenix, Arizona. It's worth noting how this particular nuclear power plant saves water. Typically, nuclear power needs a substantial amount of freshwater usage as makeup water due to the loss of water via the cooling towers. But in this case, that freshwater use is reduced by instead utilizing wastewater from the west side of the city as the cooling water to the plant. As a result, the concept of using nuclear power has become part of freshwater conservation efforts by utilizing wastewater reuse!
But water creation, treatment and delivery by utilities requires a lot of water as well. Desalination is a solution that is gaining more and more interest, especially in water-starved Western U.S. regions.
However, desalination needs a lot of energy to work. In one of my 2022 LinkedIn posts, I detailed the high energy usage of desalination. To serve 400,000 people 50 gallons of water per day per person, it would take about 650,000 kWh, which equated to 1.6 kWh per person to create this water. Considering the average home refrigerator uses 1-2 kWh per day, I asked the question, “Assuming no new energy sources, if desalination is used to create water for you, would you choose water or your refrigerator?” A tough choice indeed. Additionally, even after traditional or innovative water treatment options, delivering water to end users still requires energy, and lots of it:
Around Las Vegas, in order to treat and deliver 1,000 gallons of water to each customer, it takes 7 kWh per customer; and
The Central Arizona project is one of the most energy-intensive water delivery systems in the country, as it requires 2.8 million MWH to move about 1.6 million acre-feet of water. This equates to about 186,286 kWh per 1,000 gallons.
The water-energy nexus is also impacted by the built environment. I regularly speak about how right-sizing plumbing systems lowers energy consumption (such as IAPMO’s Water Demand Calculator for all domestic water, using engineering principles as outlined in ASPE’s Engineering Methodologies to Reduce the Risk of Legionella in Premise Plumbing Systems for hot water return system design and sizing). Heat pump water heaters are another technology that is gaining market share quickly in the U.S. because of the potential to lower energy usage by potable water systems. Expect to see more efforts to make domestic water systems more efficient to promote energy conservation.
Not all trends in the built environment will lead to energy reduction, though, and often for good reason. Water reuse is becoming a hot-button item in buildings, as consideration of these water-conserving systems is increasing. But sometimes, the “safety/sustainability nexus” shows the necessity of prioritizing safety over sustainability: The 2024 Uniform Plumbing Code (UPC) includes a new provision giving design professionals the needed language to priority public health and safety over energy efficiency when necessary. Since 2015, the International Energy Conservation Code (IECC) has included provisions to turn off hot water return pumps when the design temperature is met. Unfortunately, this can lead to hydraulically remote sections of piping not reaching design temperature (especially if the system is unbalanced). The 2024 UPC includes language exempting health care and hospitality facilities from shutting off the return pump. During the technical committee meetings, these two occupancy types were targeted because they had the highest number of Legionnaires’ disease cases. While the technical committee commented on this potential correlation issue between the codes, ultimately, the provision was added to show leadership in the realm of public health and safety.
Finally, the idea that domestic hot water systems can act as an energy storage (i.e., acting as a battery) is worth addressing. Unfortunately, at this time, safety and the limits of digitalization in plumbing systems make the likelihood of this far off. In part, this is because most plumbing systems are not sophisticated enough to handle extreme temperature fluctuations, making it difficult to prevent scalding while temperatures in systems increase drastically.
Case in point, I had the privilege of speaking with Wanda Lau at the Building Innovation Conference in Washington, D.C., in October. In her presentation, Wanda discussed the use of smart building technology. The highest adoption was by HVAC and electrical systems. The lowest? Plumbing and irrigation. More worrying was the fact that in terms of implementation, plumbing ranked at a lower priority than irrigation moving forward. Without smart technology, plumbing systems won’t have the ability to safely store excess energy.
I knew that three of our retrofit hydronic makeovers had been carefully tracked by mechanical engineers regarding their energy consumption before and after while utilizing heating degree-day information for an apples-to-apples comparison. I knew how much we had lowered the LWT temperatures and while Trethewey’s 3:1 ratio was not precise, it was darn close to being spot-on.
Agriculture water use: Smarter farming and smarter development
I would be remiss if I didn’t at least touch on agricultural water use, especially considering that it accounts for another 40% of water usage. Some of the biggest concerns of agricultural water use are coming out of my home region, the West. Unfortunately, it seems that much of it is self-inflicted. Arizona is leasing farmland to a Saudi water company, which is overusing water and threatening future water supply in Phoenix. Why? To grow the very water-intensive alfalfa to feed its cows in the Middle East. But it wouldn’t be fair to blame outside countries for our water problems — we as a nation have been incredibly shortsighted in feeding our own. Take, for example, the National Geographic documentary “Water and Power: A California Heist,” where avocado and almond farming, which are also incredibly water intensive, dominate agriculture fields. It takes 60 gallons of water to make one avocado while it takes over 1 gallon of water to make one small almond. It has gotten to the point in many areas of California where freshwater sources in these areas have dried up to the point that the local residents have to truck in their freshwater and fill water storage tanks located in their front or backyards. This is all because the ground water wells are drying up to do overuse by the farming. We simply must get smarter about which crops we grow where, and what we eat.
Worth emphasizing here is the word, “smarter.” I am not proposing that we eliminate or ban these crops. Heavy-handed, one-size-fits-all approaches can cause unintended consequences. Additionally, we need to realize that agriculture impacts water quality, as fertilizers are made with ammonia nitrates. The nitrates can make their way into the soil and penetrate aquafers. It is important to note that “elevated nitrate/ nitrite levels are typically associated with elevated levels of total coliform, E. coli., herbicides, pesticides, waterborne pathogens and more.”
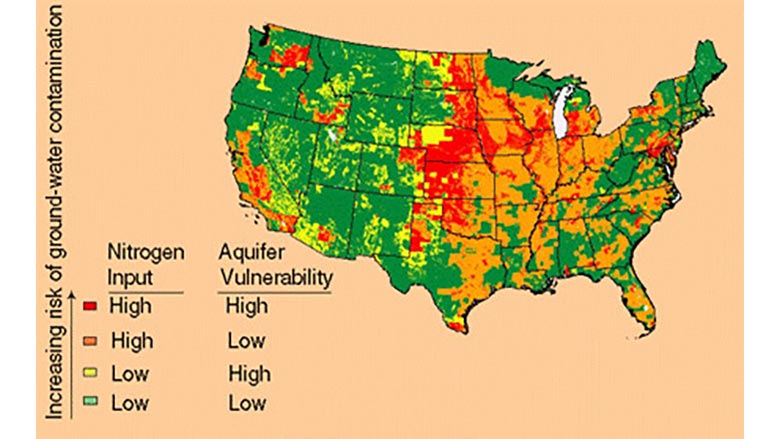
Even with the concern posed by fertilizer, banning it is also not a solution. Case in point is when fertilizer was banned in Sri Lanka, forcing all the farmers to go organic. The result? A nation was ruined due to not having enough food. Inflation reached over 50%. Nine in 10 Sri Lankan families skipped meals. The production of tea — for which Sri Lanka is famous — fell by 18%. Rice production dropped 2%, causing the causing the country to have to import rice. Synthetic fertilizers like ammonia nitrate make crops grow faster and bigger than organic fertilizer, and help millions of people, like those in Sri Lanka, get out of poverty and prevent malnutrition.
In other parts of the world, like in Arizona, replacing farmland with urban environment may end up being an inadvertent (or intentional?) water-conserving measure. I recently attended a town hall put on by Phoenix Water discussing water conservation, and also listened to a YouTube video podcast with Arizona State University Water Policy Expert Kathryn Sorensen, both of which discussed the 20% reduction in Colorado River Water to Arizona. While there is concern, the overarching message is one of opportunity. Through these events, I learned one very surprising fact: Even though Phoenix’s population has doubled, overall water use has decreased. The primary driver: Agriculture uses more water per acre than urban environments. So while more people moved to the Valley, the city became more water efficient by trading farmland with home developments (especially ones with minimal grass). The golf courses, though, are a different story — but that’s one for another time.
Summary
This series started by talking about Roy Hunter, and we ended up talking about the water-energy nexus and agriculture. We need to remember that everything we do is linked. We don’t work in a vacuum; we work in an ecosystem. In this ecosystem, each component interacts with another component. Unfortunately, we engineers are detail oriented and often can’t see the forest for the trees. We need to remember to take a step back and look at the bigger picture. Doing so will help us prevent unintended consequences from happening.
We need to remember that water is energy intensive. For us to have water, we need cost-effective, dense and reliable energy sources. Plumbing systems require energy. For safety reasons, and based on current technological limitations, plumbing system energy reduction should be evaluated with extreme scrutiny. Finally, we need to take a systems approach when evaluating water conservation and energy reduction, both within the building and without. Only this type of holistic evaluation will allow us to make the best design happen inside the building.
Editor’s Note: This column is part of a series. Catch up with Part 1, Part 2, Part 3, and Part 4.
Looking for a reprint of this article?
From high-res PDFs to custom plaques, order your copy today!